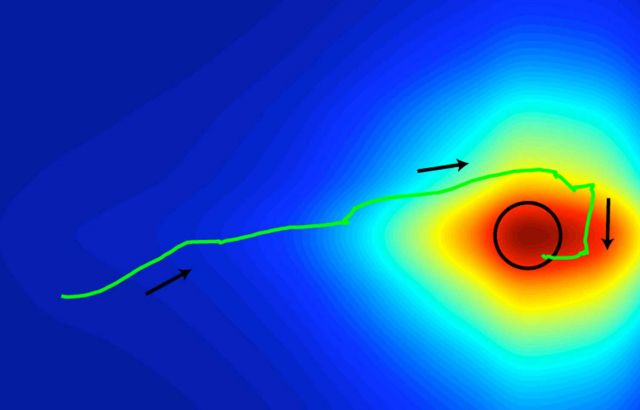
Neural Computations Underlying Sensorimotor Integration
Combining electrophysiology, optogenetics, quantitative behavioral experiments and computational modeling, we study how dynamical olfactory signals experienced during unconstrained behavior in odor gradients are processed by first-order sensory neurons of the Drosophila larva, and how this information is converted into navigational behavior. The activity of a single larval olfactory sensory neuron (OSN) is sufficient to direct larval chemotaxis. By characterizing the activity of a targeted OSN in response to naturalistic changes in stimulus intensity, we reported the surprising degree of information processing taking place in first-order olfactory neurons. Using an ordinary-differential-equation (ODE) formalism, we found that the nonlinear response characteristics of an OSN can be described by a general class of regulatory motifs that includes the incoherent feedback loop and the integral feedback. This quantitative model explains two basic operations carried out by the OSN on the stimulus: temporal differentiation on short timescales and temporal integration on longer timescales, which in concert enable the OSN to detect features related to changes in the stimulus acceleration. Using optogenetics in virtual reality experiments, we examined the sensorimotor integration underlying the navigation of odor gradients. We investigated how increases in the activity of an OSN can suppress turning, whereas abrupt decreases in activity can initiate turning. The model explains how the conversion of OSN activity into a choice between alternative behavioral states — running or turning — can be accounted for by a simple stochastic model. The overarching goal of this project is to clarify the link between neural computations at the sensory periphery and action selection during goal-oriented navigational behaviors.