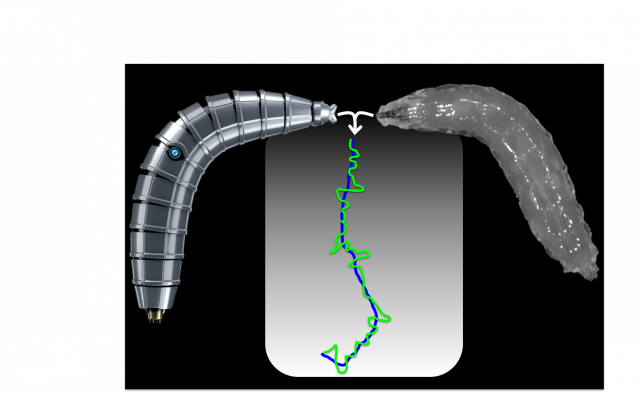
Philosophy
Neuroscience is in a state of exponential growth. Experiments that were simply unthinkable two decades ago have now become feasible or routine. For instance, the activity of single genetically-targeted neurons can be monitored and manipulated, which opens a path to crack the function of neural circuits. Machine-learning algorithms and artificial intelligence permit the decomposition of complex behaviors into subsets of stereotyped motor programs. Meanwhile, genome editing has blurred the traditional divide between genetic and non-genetic model organisms. While these technical progress are creating extraordinary research opportunities, they can also be intimidating in their outcomes. Big datasets are quickly piling up in the lab. The profusion of novel reagents is such that the number of possible experiments one can conduct greatly exceeds the timescale of any PhD or any post-doc. What is the research philosophy of our lab to navigate a field in constant revolution?
As neuroethologists, we let animals show us the way. To paraphrase Dobzhansky, we believe that "nothing in neuroscience makes sense except in light of behavior.” The starting point of our research is a careful inspection of innate behaviors. This analysis points out key problems that an animal must solve to survive in the wild. Over the years, work from our lab has established that chemotaxis in the Drosophila larva is surprisingly precise and reproducible (it is “robust”). Therefore, the stereotypy of this behavior favors our attempt to break complex phenomena into elementary mechanisms controlled by neural circuits in the brain. To this end, what research strategy are we following?
We adopt a quantitative Biology (qBio) approach to characterize the neural computations underlying navigation behavior. Guided by the idea that technology is an engine that empowers experimentation, we have developed new methodologies to study behavioral responses in response to olfactory stimuli that can be measured and controlled. Combined with closed-loop tracking, we exploit optogenetics to produce virtual olfactory realities (fictive odor stimulation evoked by light). Experimental data are evaluated in light of generic models of sensory coding (perception) and sensory-to-motor control. Rather than being a goal per se, we view computational modeling as a tool to derive and test mechanistic hypotheses. Our conceptual understanding advances based on the iteration of a loop between experimentation and theory. While the predictions and limitations of a model direct the design of new experiments at the bench, new “wet” data leads to the refinement of “in silico” model. But, how do our lab members take on the challenge of working at the interface between disciplines?
Our lab is actively deconstructing traditional boundaries between Biology, Physics and Bioengineering. We are interdisciplinary to the core. Most of our lab members have a background in different disciplines. Irrespective to this difference in background, every lab member is trained to work at the interface between the disciplines defining a project. We believe that it is essential for an experimentalist to acquire the computational skills to see through a big dataset and to evaluate the predictions and limits of a model. Conversely, we believe that it is essential for a theorist to gain first-hand experience of the system being modeled. A major goal of our lab is to create an environment in which experimentalists can become familiar with theory and theorists can be immersed into experimentation. This cross-training is essential to initiate a fruitful dialogue between individuals who have complementary views on Neuroscience. Another goal of our lab is to foster individuals to express their creativity and use collaboration to fulfill their research potentials.
While neuroscience is in a state of constant mutation, our methodology keeps adapting to push the realm of the possible. And yet our destination remains invariant: we aim to answer one of the oldest questions that has kept scientists and philosophers busy … how do brains work? The pace at which our mechanistic understanding of brain functions has progressed in Drosophila is stunningly fast. So much ground can be covered in just 4 or 5 years. Whether you are a student or a post-doc, come and join our efforts to explain the neural computations directing behavior in a mini brain.